Discover the importance of conductivity sensors and meters in assessing solution quality. Learn about the principles behind conductivity measurements and how these devices ensure accurate readings, crucial for maintaining water purity, monitoring industrial processes, and upholding quality standards across various applications.
Key Technologies Driving Innovation in Conductivity Sensor
Conductivity sensors harness a myriad of technologies to deliver accurate and reliable measurements:
- Electrode Configurations: Varied electrode configurations, including two-electrode and four-electrode setups, cater to diverse application needs, balancing simplicity with precision.
- AC and DC Measurement: Alternating current (AC) and direct current (DC) measurement methodologies offer distinct advantages, allowing for stable and accurate measurements across different conductivity ranges.
- Temperature Compensation: Integration of temperature sensors ensures accurate measurements irrespective of temperature fluctuations, enhancing the reliability of conductivity readings.
- Material Selection: From stainless steel to platinum and graphite, the choice of electrode materials is tailored to suit specific application requirements, ensuring durability and performance.
- Smart Sensor Technologies: Incorporation of digital interfaces and advanced electronics empowers conductivity sensors with features like automatic calibration and real-time data logging, bolstering usability and efficiency.
Working Principle of a Conductivity Meter:
A conductivity meter serves as a cornerstone in the realm of analytical instrumentation, offering invaluable insights into the conductivity of solutions across various industries. At its heart lies a sophisticated interplay of principles and technologies, culminating in precise and reliable measurements. Let’s delve into the intricate workings of this essential device:
Electrodes Configuration:
Central to the operation of a conductivity meter are its electrodes. Typically, a conductivity meter employs two or four electrodes immersed in the solution under scrutiny. These electrodes, often made of conductive materials like stainless steel or platinum, play a pivotal role in facilitating the flow of electric current through the solution.
Electrical Potential Application:
Upon immersion in the solution, a conductivity meter applies an electrical potential (voltage) across the electrodes. This applied voltage generates an electric field within the solution, setting the stage for the movement of ions.
Ion Movement:
In a solution, various ions (charged particles) may be present, contributing to its conductivity. When the electrical potential is applied, these ions begin to migrate towards oppositely charged electrodes. Positively charged ions, known as cations, move towards the negatively charged electrode (cathode), while negatively charged ions, or anions, gravitate towards the positively charged electrode (anode).
Current Flow:
As ions traverse the solution towards their respective electrodes, they carry electric charge, thus facilitating the flow of electric current. The magnitude of this current flow is directly proportional to the conductivity of the solution—higher conductivity corresponds to greater current flow.
Measurement and Calculation:
The conductivity meter meticulously measures the electric current flowing between the electrodes. Leveraging Ohm’s Law (I = V/R), where I represents the current, V denotes the voltage, and R signifies the electrical resistance, the conductivity of the solution is calculated. This conductivity value, often expressed in Siemens per meter (S/m) or microsiemens per centimeter (μS/cm), serves as a quantitative indicator of the solution’s ability to conduct electricity.
Temperature Compensation:
Recognizing the temperature dependency of conductivity, advanced conductivity meters incorporate temperature compensation mechanisms. These mechanisms ensure that variations in temperature do not unduly influence the accuracy of conductivity measurements, thereby enhancing the reliability of the device across diverse operating conditions.
Calibration and Validation:
To maintain accuracy and precision, conductivity meters undergo periodic calibration against standard reference solutions with known conductivity values. Additionally, validation procedures, including regular performance checks and quality assurance measures, are employed to uphold the integrity of measurement results.
User Interface and Data Output:
Modern conductivity meters are equipped with user-friendly interfaces and digital displays, allowing operators to input parameters, monitor measurements in real-time, and access stored data. Some advanced models may also feature data logging capabilities, enabling the recording and analysis of conductivity trends over time.
In essence, the working principle of a conductivity meter revolves around the meticulous measurement of electric current in a solution, elucidating its conductivity with precision and accuracy. By harnessing the innate properties of ions and electric fields, conductivity meters empower industries and researchers alike with invaluable insights into the composition and behavior of solutions, paving the way for innovation and discovery.
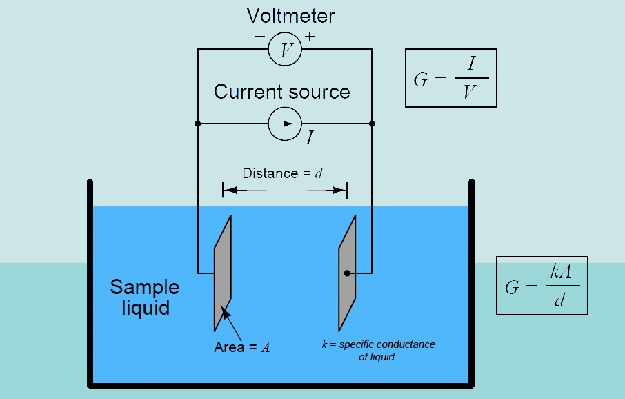
Conductivity sensor types:
Conductivity sensors come in various types, each designed for specific applications and environments. Here are some common types of conductivity sensors:
- Contacting Conductivity Sensors:
- Two-Electrode Sensors: Simple design with two electrodes immersed in the solution, suitable for basic conductivity measurements in clean liquids.
- Four-Electrode Sensors: More advanced design with additional electrodes to minimize polarization and improve accuracy, ideal for demanding applications with high conductivity or conductivity changes.
- Inductive Conductivity Sensors:
- Inductive sensors use electromagnetic induction to measure conductivity without direct contact with the solution. They are suitable for applications where sensor fouling or corrosion is a concern.
- Toroidal Conductivity Sensors:
- Toroidal sensors feature a toroidal (doughnut-shaped) design that surrounds the solution without contacting it directly. They provide accurate measurements in harsh or corrosive environments and are less prone to fouling compared to contacting sensors.
- Flow-Through Conductivity Sensors:
- Flow-through sensors are integrated into flow cells or pipelines, allowing continuous measurement of conductivity in flowing liquids. They are commonly used in industrial processes and water treatment systems.
- Submersible Conductivity Sensors:
- Submersible sensors are designed to be immersed directly into the solution for continuous monitoring. They are widely used in environmental monitoring, water quality assessment, and aquaculture applications.
- In-Line Conductivity Sensors:
- In-line sensors are installed directly in a pipeline or process line, providing real-time conductivity measurements during fluid flow. They are suitable for industrial processes requiring continuous monitoring and control.
- Differential Conductivity Sensors:
- Differential sensors measure the difference in conductivity between two points in a solution, allowing for more precise measurements and compensation for temperature and pressure variations.
- Smart Conductivity Sensors:
- Smart sensors incorporate digital interfaces and advanced electronics for enhanced functionality, such as automatic calibration, data logging, and communication with external devices. They offer greater convenience and accuracy in conductivity measurement applications.
Conductivity relation with temperature:
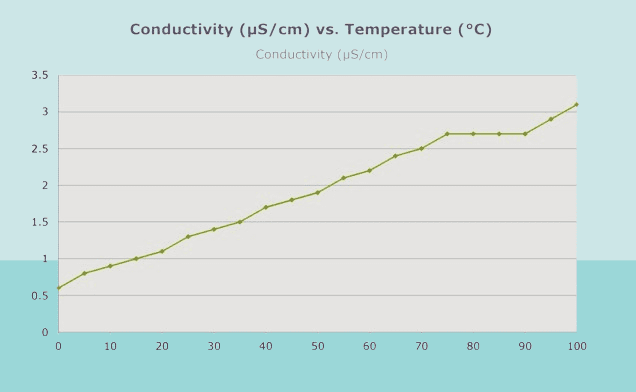
Conductivity is directly influenced by temperature, and it’s essential to consider temperature effects when measuring conductivity. Here’s how conductivity relates to temperature:
- Temperature Coefficient of Conductivity (α): Conductivity typically increases with temperature. The relationship between conductivity and temperature is described by the temperature coefficient of conductivity (α), which quantifies the change in conductivity per degree change in temperature. The α value varies depending on the solution’s composition and is typically provided by the manufacturer for calibration purposes.
- Effect on Ionic Mobility: As temperature rises, ions in the solution gain more kinetic energy, leading to increased mobility. This increased mobility allows ions to move more freely through the solution, resulting in higher conductivity.
- Temperature Compensation: To account for temperature effects on conductivity measurements, conductivity meters often include temperature compensation mechanisms. These mechanisms adjust conductivity readings based on the solution’s temperature to provide accurate and consistent measurements regardless of temperature variations.
- Thermal Conductivity of the Solution: Temperature can also affect the thermal conductivity of the solution, influencing the overall conductivity measurement. Changes in solution density, viscosity, and ion dissociation can further impact conductivity with temperature variations.
- Standardization: When calibrating conductivity meters or conducting conductivity measurements, it’s crucial to use standardized procedures and reference solutions at specific temperatures. This ensures accurate comparisons and reliable conductivity values across different temperature conditions.
In summary, conductivity is intimately linked to temperature, with conductivity typically increasing as temperature rises. Understanding and compensating for temperature effects are essential for obtaining accurate and reliable conductivity measurements in various applications.
Design formulas used by conductivity meter
Conductivity sensors or meters typically measure the conductivity of electrolyte solutions, which contain ions that facilitate the flow of electrical current. Here are some common chemical formulas and technical calculations relevant to conductivity measurements:
1. Electrical Conductivity (σ):
- Formula: σ = κ * A / L
- Description: Electrical conductivity (σ) is the ability of a substance to conduct an electric current. It is calculated based on the conductivity (κ) of the solution, the area (A) of the electrodes, and the distance (L) between them.
2. Specific Conductance (κ):
- Formula: κ = G * (L / A)
- Description: Specific conductance (κ) is a measure of the conductivity of a solution. It is calculated based on the conductance (G) of the solution, which is the reciprocal of the resistance measured by the conductivity sensor. The area (A) and distance (L) between the electrodes are also considered.
3. Conductance (G):
- Formula: G = 1 / R
- Description: Conductance (G) is the reciprocal of electrical resistance (R). It represents the ease with which an electric current passes through a solution and is measured in siemens (S).
4. Concentration of Ions (C):
- Formula: C = σ / (z * F)
- Description: The concentration of ions (C) in a solution can be calculated based on the electrical conductivity (σ), the charge of the ion (z), and Faraday’s constant (F), which represents the charge per mole of electrons.
5. Ion Mobility (μ):
- Formula: μ = σ / (C * z)
- Description: Ion mobility (μ) is a measure of the speed at which ions move through a solution under the influence of an electric field. It is calculated based on the electrical conductivity (σ), the concentration of ions (C), and the charge of the ion (z).
6. Temperature Compensation:
- Formula: κ(T) = κ(25°C) * [1 + α(T – 25°C)]
- Description: Conductivity measurements are often temperature-dependent. To compensate for temperature variations, a correction factor α is applied based on the difference between the measured temperature (T) and a reference temperature (usually 25°C).
7. Calibration Factor (CF):
- Formula: CF = Actual Conductivity / Measured Conductivity
- Description: The calibration factor (CF) is used to adjust conductivity measurements to match the actual conductivity of a solution. It is calculated by dividing the actual conductivity by the conductivity measured by the sensor.
Applications of the Conductivity Sensor / Conductivity Meter
Application | Description |
---|---|
Water Quality Monitoring | Assessing the purity of drinking water, evaluating effectiveness of wastewater treatment processes. |
Industrial Processes | Monitoring ion concentrations in chemical processing industries, ensuring product quality in food and beverage production. |
Environmental Monitoring | Measuring salinity levels in aquatic ecosystems, assessing soil salinity in agriculture. |
Laboratory Research | Analyzing ion concentrations in chemical solutions, studying biomolecule behavior in biochemical research. |
Cooling Systems | Monitoring water quality in cooling towers, preventing scale formation and corrosion. |
Quality Control in Manufacturing | Ensuring quality standards in electronics manufacturing, maintaining precise conditions in pharmaceutical production. |
Power Plants | Monitoring boiler water quality to prevent scale formation, optimizing heat transfer efficiency. |
Biotechnology | Monitoring and controlling fermentation processes, ensuring optimal conditions for microbial growth. |
How to calibrate the Conductivity Meter ?
Calibrating a conductivity meter in a pharmaceutical plant is crucial to ensure accurate and reliable measurements, which are essential for maintaining quality standards in pharmaceutical production. Here’s a step-by-step guide on how to calibrate a conductivity meter in a pharmaceutical plant:
1. Preparation:
- Gather the necessary equipment, including calibrated conductivity standards (often provided by the meter manufacturer), a clean beaker or container, and gloves to maintain cleanliness.
- Ensure the conductivity meter is clean and free from any residue or contaminants.
2. Selection of Calibration Standards:
- Choose the appropriate calibration standards based on the expected conductivity range of the solutions to be measured.
- It’s common to use at least two standard solutions: a low-conductivity standard and a high-conductivity standard. These solutions should bracket the expected range of conductivity measurements in the pharmaceutical process.
3. Calibration Procedure:
- Start by rinsing the conductivity cell of the meter with deionized or distilled water to remove any residues.
- Fill a clean beaker or container with the first calibration standard solution, ensuring there is enough solution to immerse the conductivity cell completely.
- Turn on the conductivity meter and allow it to stabilize according to the manufacturer’s instructions.
- Immerse the conductivity cell into the calibration standard solution and wait for the reading to stabilize.
- Adjust the meter’s calibration settings to match the conductivity value of the calibration standard. Follow the specific instructions provided by the manufacturer for adjusting calibration settings.
- Rinse the conductivity cell with deionized or distilled water to remove any traces of the first calibration standard.
- Repeat the calibration procedure with the second calibration standard solution following the same steps as above.
- After calibrating with both standards, ensure that the meter displays the correct values for each standard solution.
4. Verification and Adjustment:
- Once calibration is complete, verify the accuracy of the meter’s readings by measuring additional standard solutions or conducting a calibration check using the same standards.
- If the meter readings deviate from the expected values, recalibrate the meter or adjust the calibration settings as necessary.
5. Documentation:
- Record the calibration process, including the date, calibration standards used, and any adjustments made to the meter’s settings.
- Maintain calibration records as part of the plant’s quality assurance documentation to demonstrate compliance with regulatory requirements.
6. Routine Calibration Checks:
- Conduct routine calibration checks at regular intervals, as specified by the plant’s standard operating procedures or regulatory guidelines.
- Ensure that calibration standards are stored properly and replaced when expired or contaminated.
By following these steps, pharmaceutical plant operators can effectively calibrate conductivity meters to ensure accurate and reliable measurements, thereby upholding quality standards in pharmaceutical production. It’s essential to adhere to good laboratory practices and regulatory requirements throughout the calibration process.
Frequently asked questions:
What is a digital conductivity meter?
Answer: A digital conductivity meter is an electronic device used to measure the electrical conductivity of a solution. It provides digital readouts of conductivity values and often includes features such as temperature compensation, data logging, and calibration options.
What sensors are used to measure conductivity?
Answer: Sensors used to measure conductivity typically consist of two or more electrodes made of conductive materials, such as stainless steel or platinum. These electrodes are immersed in the solution, and the conductivity is measured based on the electrical properties of the solution.
What is conductivity in a conductivity meter?
Answer: Conductivity, in the context of a conductivity meter, refers to the ability of a solution to conduct an electrical current. It is a measure of the concentration of ions present in the solution, with higher conductivity indicating a higher concentration of ions.
How do you test conductivity with a conductivity meter?
Answer: To test conductivity with a conductivity meter, immerse the electrodes of the meter into the solution to be tested. The meter will measure the electrical conductivity of the solution and display the result in units such as Siemens per meter (S/m) or microsiemens per centimeter (μS/cm).
What is the principle of conductivity?
Answer: The principle of conductivity is based on the ability of ions in a solution to carry an electrical current. When electrodes are immersed in a solution, ions move towards oppositely charged electrodes, facilitating the flow of current, which is measured to determine the solution’s conductivity.
How is conductivity measured?
Answer: Conductivity is measured by immersing electrodes in a solution and applying a voltage across them. The resulting current flow is measured, and conductivity is calculated using Ohm’s Law (I = V/R), where I is the current, V is the voltage, and R is the electrical resistance.
What is the unit of conductivity?
Answer: The unit of conductivity is Siemens per meter (S/m) in the International System of Units. It can also be expressed in microsiemens per centimeter (μS/cm).
How do conductivity sensors work?
Answer: Conductivity sensors work by applying a voltage across electrodes immersed in a solution. The resulting current flow is measured, and conductivity is calculated based on the relationship between current, voltage, and electrical resistance.
Where is a conductivity meter used?
Answer: A conductivity meter is used in various industries and applications, including water quality monitoring, industrial processes, environmental monitoring, laboratory research, and quality control in manufacturing.
Why is conductivity used?
Answer: Conductivity is used as a measure of the concentration of ions in a solution, providing valuable information about its chemical composition and purity. It is essential for ensuring water quality, monitoring industrial processes, and conducting scientific research.
How do you test a conductivity sensor?
Answer: To test a conductivity sensor, you can use standardized conductivity solutions of known conductivity values. Immerse the sensor in the solution and compare the reading with the known value to determine the accuracy of the sensor.
What is an example of conductivity?
Answer: An example of conductivity is the ability of saltwater to conduct electricity due to the presence of dissolved ions such as sodium and chloride.
What is the symbol for conductivity?
Answer: The symbol for conductivity is σ (sigma).
What is a simple definition of conductivity?
Answer: Conductivity is the measure of a material’s ability to conduct an electric current, typically in the form of ions in a solution.
What is the output of a conductivity sensor?
Answer: The output of a conductivity sensor is typically a numerical value representing the electrical conductivity of the solution being measured, often expressed in units such as Siemens per meter (S/m) or microsiemens per centimeter (μS/cm).
What is the working principle of a conductivity meter?
Answer: The working principle of a conductivity meter is based on applying a voltage across electrodes immersed in a solution, measuring the resulting current flow, and calculating the conductivity of the solution based on Ohm’s Law.
What is the function of a digital conductivity meter?
Answer: The function of a digital conductivity meter is to accurately measure the electrical conductivity of a solution and provide digital readouts of conductivity values, often including features such as temperature compensation, data logging, and calibration options.
How does a conductivity sensor work?
Answer: A conductivity sensor works by applying a voltage across electrodes immersed in a solution. The resulting current flow is measured, and conductivity is calculated based on the relationship between current, voltage, and electrical resistance.
What are the applications of a conductivity sensor?
Answer: Conductivity sensors are used in various applications, including water quality monitoring, industrial processes, environmental monitoring, laboratory research, and quality control in manufacturing.
What is the formula of a conductivity sensor?
Answer: The formula for conductivity measurement using a conductivity sensor is typically based on Ohm’s Law (I = V/R), where I is the current, V is the voltage, and R is the electrical resistance, with conductivity (σ) calculated from these values.
I sincerely enjoyed what you have produced here. The design is refined, your authored material trendy, yet you appear to have obtained a degree of apprehension regarding what you aim to offer next. Certainly, I shall return more frequently, just as I have been doing almost constantly, provided you uphold this incline.